Synergy Of PX and SMX on One Diffractometer with the HyPix-Arc 150°
Introduction
Small molecule and macromolecular crystallographers use the same technique, often work in close proximity, and sometimes collaborate on the same projects, yet they are mostly separate in practice, many times even using separate diffractometers in the same room. Our newest diffractometers were designed around the concept of synergy of techniques. Here, we use our newest hybrid photon counting detector, the HyPix-Arc 150°, to demonstrate this synergy. We use the same instrument to collect atomic resolution data sets of lysozyme with and without sucrose bound and of sucrose by itself. We also solve all three structures by experimental phasing.
Experimental overview
Orthorhombic hen egg-white lysozyme crystals were grown at 18°C by vapor diffusion using 50 mg/mL lysozyme mixed 1:1 with crystallization solution [0.1 M NaOAc, pH 4.4, 0.1 M NaCl, 0.5 M (NH₄)₂SO₄, 10 mM KAu(CN)₂] suspended over a well of 0.5 mL crystallization solution. For crystals with sucrose bound, 30% (w/v) sucrose was included in the crystallization solution (C. Fan, personal communication, February 2020). Lysozyme crystals were swished through 20% ethylene glycol for cryoprotection. The sucrose crystal used was cut from a large, single turbinado sugar crystal under Parabar 10312 oil. All crystals were mounted on a Rigaku XtaLAB Synergy-Custom and collected at 100 K.
Figure 1: Crystals of lysozyme (top left), lysozyme + sucrose (top right), and sucrose (bottom) used here. The gridlines are 0.1 mm apart.
Table 1: XtaLAB Synergy-S specifications.
X-ray source | MicroMax-007 HF Cu |
Operating power | 40 kV x 30 mA = 1.2 kW |
X-ray optic | Confocal VariMax VHF |
Beam characteristics | FWHM = 100 μm, Divergence = 10 mrad |
Goniometer / Detector range | 4- circle Kappa with telescoping 2θ arm / distance range of 35 – 210 mm |
Detector Active area Readout time Pixel size Cooling |
Hybrid photon counting HyPix-Arc 150° 77.5 x 121.8 mm Up to 70 Hz 100 µm water-cooled |
Results
The crystallization conditions used here are intended to yield orthorhombic lysozyme crystals that can be solved by Au-SAD phasing. A lysozyme crystal (116 μm x 135 μm x 235 μm) grown without sucrose (LY; Figure 1) was mounted and screened on the XtaLAB Synergy-Custom using CrysAlisPro in PX mode. Diffraction spots were visible to 1.18 Å (Figure 2), so a strategy to collect a complete data set to a resolution limit of 0.9 Å was calculated by CrysAlisPro and collected in ~2 hours (Table 2). Then, a larger lysozyme crystal (277 μm x 355 μm x 412 μm) grown with sucrose (LY+sucrose; Figure 1) was mounted and screened (Figure 3). The highest-resolution diffraction spots were observed near 0.96 Å, so a strategy to collect a complete data set to a resolution limit of 0.85 Å was calculated by CrysAlisPro and collected in ~36 minutes (Table 2).
Figure 2: A 2-second exposure per 0.2° rotation of the LY crystal at distance of 41 mm and 2° of 55.26°.
Figure 3: A 0.6-second exposure per 0.2° rotation of the LY+sucrose crystal at distance of 41 mm and 2° of 60.37°.
Results
The data sets were processed and scaled by CrysAlisPro, exported to an unmerged mtz file, and then merged with AIMLESS¹. Both data sets were cut at a resolution limit where the mean signal-to-noise ratio remained above 1 and the CC½ was ~0.5 (Table 3). For LY, these criteria resulted in a 0.97 Å data set with over 7-fold redundancy and a few percent difference in the Rmerge versus the Rmerge anomalous—as expected due to the strong Au anomalous signal. For the LY+sucrose, a 0.87 Å data set was achieved with 7-fold redundancy and a similar difference in the merging R factors.
Table 2: Data collection parameters for all crystals.
LY | LY+sucrose | Sucrose | |
Total # images | 3376 | 3760 | 2572 |
Total # scans | 18 | 17 | 25 |
Total data collection time | 1h 56m 34s | 36m 8s | 32m 22s |
Both structures were solved using programs from the CCP4i² interface. First, SHELXC/D/E³ was used to identify the substruc-ture of Au, S, and Cl atoms, generate initial phases, and identify the correct hand of the solution. Then, DM⁴ was used for density modification and Buccaneer⁵ was used to build the initial model. Each model was completed by iterative cycles of manual model building in Coot⁶ followed by restrained refinement with Refmac⁷. The B factors were refined anisotropically. The models were finalized after one round of validation using the PDB_REDO⁸ webserver.
Table 3: Data processing and refinement results
LY | LY+sucrose | |
Space group | P2₁2₁2₁ | P2₁2₁2₁ |
Unit cell | 30.0 Å, 55.9 Å, 72.8 Å | 30.0 Å, 56.0 Å, 71.6 Å |
Resolution (last shell) | 24.8 – 0.97 Å (0.99–0.97 Å) | 23.0 – 0.87 Å (0.88–0.87 Å) |
Completeness (last shell) | 100% (100%) | 100% (100%) |
Redundancy (last shell) | 7.6 (5.4) | 7.0 (4.3) |
<I/σI> (last shell) | 16.9 (1.5) | 19.6 (1.2) |
Rmerge (last shell) | 7.5% (123.8%) | 5.8% (106.8%) |
Rmerge anomalous (last shell) | 5.7% (110.2%) | 3.7% (94.5%) |
CC½ | 1.0 (0.5) | 1.0 (0.45) |
Rwork | 13.1% | 12.5% |
Rfree | 14.2% | 13.2% |
The catalytic pocket of the LY structure (in the vicinity of Asp52) was largely empty with some water molecules and a single molecule of ethylene glycol (Figure 4). The LY+sucrose active site had perfectly clear electron density for a single molecule of sucrose with no obvious disorder (Figure 5).
Figure 4: Active site of LY. Maps shown are 2Fo-Fc in gray (@3.5 rmsd), positive Fo-Fc in green (@4 rmsd), and negative Fo-Fc in red (@-4 rmsd).
Figure 5: Active site of LY+sucrose. Maps shown are 2Fo-Fc in gray (@4 rmsd), positive Fo-Fc in green (@4.5 rmsd), and negative Fo-Fc in red (@-4.5 rmsd).
Figure 6: A summation of 20 diffraction images (10° of rotation) for the sucrose crystal at a distance of 41 mm and 2° of 73°. Each image was 0.25-second exposure per 0.5° rotation
Data processing in CrysAlisPro yielded a completeness of 99.8%, an Rint of 2.0%, and a mean signal-to-noise ratio of 67 (Table 4). The structure was solved in Olex2⁹, using SHELXT¹⁰ for structure solution and SHELXL¹⁰ for refinements (Figure 7). Hydrogen atoms were placed as riding atoms. Excellent structural statistics were obtained, with R1 at 2.33% and goodness of fit at 1.049.
Table 4: Data processing and refinement results for sucrose
Space group | P2₁ |
Unit cell | 7.7207(2) Å, 8.6690(3) Å, 10.8139(3) Å 90º, 102.985(3)º, 90º |
Resolution (last shell) | 10.54–1.86 Å (0.87–0.84 Å) |
Completeness (last shell) | 99.8% (98.6%) |
Redundancy (last shell) | 5.4 (3.0) |
<I/σI> (last shell) | 67.1 (22.3) |
Rint (last shell) | 2.0% (5.1%) |
Final R factors [1>2σI] | R1 = 2.33% wR2 = 5.80% |
Goodness of fit | 1.049 |
Flack | 0.04(7) |
Largest residual peak/hole | 0.16 eų / -0.20 eų |
Figure 7: View of the final model for sucrose.
Conclusion
Here, a set of three crystal samples was used to loosely represent an enzyme target (LY), a small molecule inhibitor (sucrose), and an enzyme-inhibitor complex (LY+sucrose). One modern Rigaku X-ray diffractometer, the XtaLAB Synergy-Custom with a HyPix-Arc 150° detector, can tackle all three samples easily and bridge the gap between macromolecular and small molecule crystallography. There is no need for separate instrumentation. Both small molecule and macromolecular crystallographers can inhabit the same space and learn more about each other’s techniques. Of course, one normally expects a small molecule to yield an atomic resolution structure while the macromolecule achieves a lower resolution, but in this instance, we were rewarded with high-resolution structures for all three structures.
References
- Evans P.R., Murshudov G.N. (2013). Acta Cryst. D69, 1204-1214.
- Winn M.D. et al. (2011). Acta Cryst. D67, 235-242.
- Sheldrick G.M. (2010). Acta Cryst. D66, 479-485.
- Cowtan K. (1994). Joint CCP4 and ESF-EACBM Newsletter on Protein Crystallography, 31, 34-38.
- Cowtan K. (2006). Acta Cryst. D62, 1002-1011.
- Emsley P., Lohkamp B., Scott W.G., Cowtan K. (2010). Acta Cryst. D66, 486-501.
- Murshudov G.N., et al. (2011). Acta Cryst. D67, 355-367.
- Joosten R.P., Long F., Murshudov G.N.,
Perrakis A. (2014). IUCrJ, 1, 213-220. - Dolomanov O.V., Bourhis L.J., Gildea R.J., Howard J.A.K., Puschmann H. (2009). J. Appl. Cryst. 42, 339-341.
- Sheldrick, G. M. (2015). Acta Cryst. A71, 3-8.
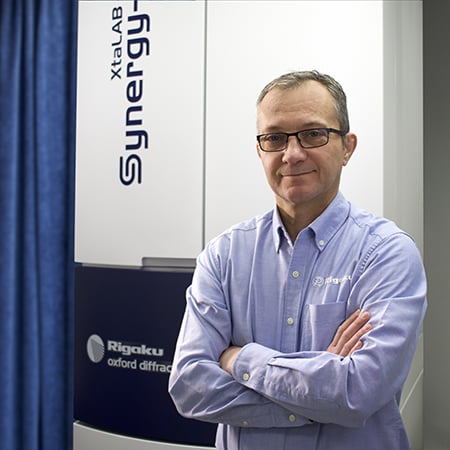
Contact Us
Whether you're interested in getting a quote, want a demo, need technical support, or simply have a question, we're here to help.